Nitrogen+Syngas 395 May-Jun 2025
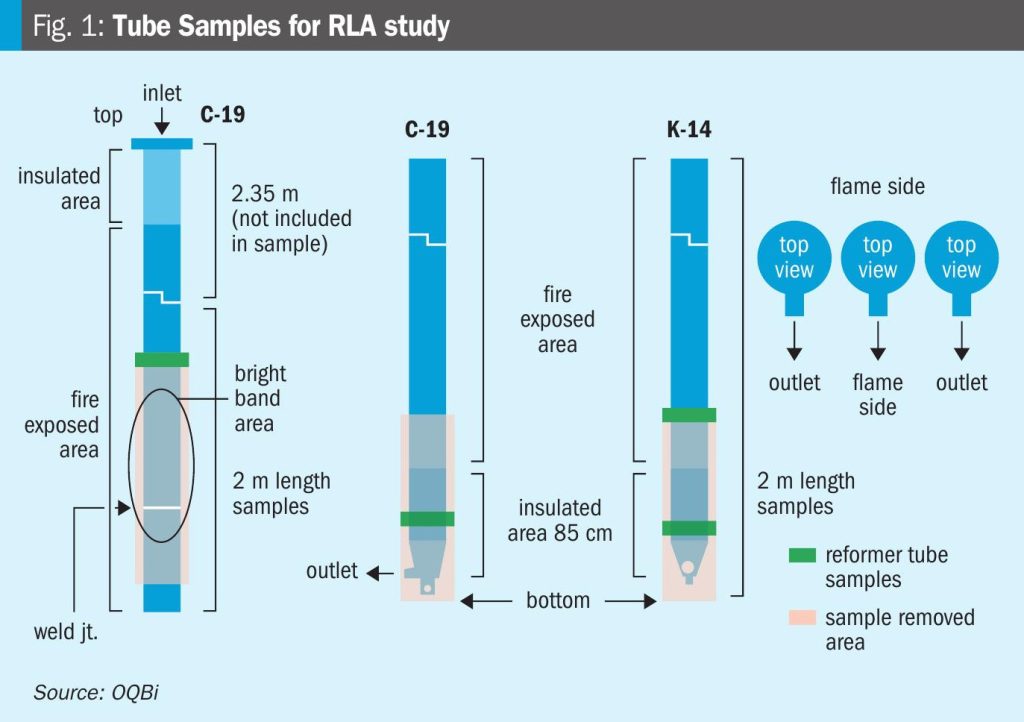
2 May 2025
Techniques to extend the life of steam methane reformer tubes
ASSET INTEGRITY
Techniques to extend the life of steam methane reformer tubes
Muhammad Faisal Faraz and Abdullah Al Balushi of OQBi explain how by implementing a series of technical and process improvements, OQBi has successfully extended the lifespan of its reformer tubes by six years beyond the original design life of 100,000 hours.
The steam methane reformer
The steam methane reformer (SMR) is a critical component for syngas production. The SMR furnace of the OQBi methanol plants is a refractory-lined, down-fired, rectangular-shaped unit with a balanced draft system utilising forced draft (FD) and induced draft (ID) fans, designed by Jacobs (Worley). The furnace contains a radiant section with 768 catalyst-filled tubes (12 rows of 64 tubes) for endothermic reactions. Heat is supplied by 234 burners arranged in 13 rows, each row containing 18 burners. The burners were replaced during a mini- turnaround in July 2021. The mixed fuel comprises natural gas from the battery limit, tail gas from the PSA unit ammonia, purge gas from the synthesis loop, and flash gas from the crude methanol flash drum. Continuous monitoring of the reformer is crucial for maintaining longterm reliability and preventing unexpected plant outages. Key parameters monitored include heat flux, tube outlet temperature, cross-header temperature, reformer outlet temperature (ROT), tube skin temperature (TST), fuel header pressure, combustion air pressure, and reformer draft.
The reformer catalyst tubes have been in service since plant commissioning and have exceeded their useful service life of 100,000 hours (~11.5 years). A life assessment performed in 2023 revealed 26 tubes with indications 2-3 m from the top, with an expected extended life of 5 to 8 years. While the tubes are projected to last until the next turnaround in 2027-2028, ensuring reliable operation within all operating limits is essential to achieve this lifespan.
Asset integrity techniques
Since steam methane reformers are considered significantly complex and critical equipment in such plants, a special focus of inspection and integrity management programs have been developed to assess the health of tubes and implemented appropriate actions to prevent any failure. In OQBi, during any opportunity, reformer catalyst tubes, outlets pigtails and manifolds/hot collectors are inspected and evaluated. The first LEO-SCAN inspection was done as a baseline in 2009 prior to reformer commissioning, followed by a second inspection in the 2014 turnaround.
In the 2018 turnaround, all 768 catalyst tubes were inspected by LEO-SCAN and ID scan. In total, 74 outlet pigtails were inspected for outside diameter (OD) growth. Due to some observed OD growth in the outlet pigtails, the temperature was revised and an integrity operating windows (IOW) program was established for the catalyst tube outlet temperatures, hot collectors/manifolds and outlet cross headers.
During a 2019 pit-stop, selected catalyst tubes were inspected (visually, in-situ metallography, hardness and permeability). In total 89 outlet pigtails were inspected for OD growth during this opportunity.
During a mini turnaround in 2021, since no major indications were observed in 2019, selectively 30% of catalyst tubes were inspected by LEO-SCAN. All outlet pigtails (768) were inspected for OD growth. Any tube which had grown above 6% in OD was replaced immediately. To revise operating parameters and assess tube integrity, a laboratory based residual life assessment (RLA) for catalyst tubes was initiated.
In the 2023 turnaround, all catalyst tubes were inspected by LEO-SCAN and ID scan. Around 30 tubes were observed with small creep expansion (<1%). Another API579-level 1 RLA was conducted based on catalyst tube OD inspection outcomes. It was concluded that, with similar average operating hours, the tubes would maintain full integrity until the next turnaround in 2028. All outlet pigtails (768) were replaced in kind during this opportunity.
The main drivers for conducting the laboratory-based remaining life assessment initiated in 2021 were:
- fuel gas variation – prior to commissioning of the LPG plant, rich gas was supplied directly to the reformer with all hydrocarbons which increased tube temperatures and hot spots; observed tube diametric growth;
• to review operating parameters to suit current tube integrity conditions;
- to assess operating over 100,000 hours as recommended by the manufacturer (manufacturer recommended to replace at 100,000 hours if the operating parameters are near to design parameters, whereas actual operating parameters were lower in average).
The RLA considered the following:
• total number of start-ups / shutdowns;
• effective operation hours;
• average operating temperature/pressure;
• historical inspection data;
• design data.
Several techniques were used to enhance the inspection and condition of the reformer tubes and pigtails.
Optical microscopy and metallographic investigation
The metallurgy of the tubes was tested (see Figs 1 and 2) and it was found that there was a significant safety margin as the reformer tubes are operating more than 100°C below the design temperature. Under such conditions no significant creep damage was anticipated.
The microstructures consisted of austenite matrix with a moderate level of the secondary carbide precipitation interspaced by interdimeric primary carbides. The level of thermal aging is moderate. There is no evidence of creep cavitation damage in the microstructure. The OD of the reformer tube contained a recrystallised layer to a depth of 0.25 mm to 0.4 mm. The ID of the reformer tube contained a decarburised layer to the depth of approximately 0.2 mm.
The reformer tubes with diametrical expansion of less than 1%, without any isolated creep cavitation in the microstructure, operate in the early secondary stage of the creep life. The estimated maximum expended life fraction is less than 0.3. The remaining life of the reformer tubes is more than 70% of the original design life. The service life of the reformer tubes is expected to be significantly greater than 100,000 hours if the current average operating temperature is maintained.
Diameter measurements and strain calculation
Based on measurements of the ‘cold section’, outside of the radiant fired section, the original OD is likely to be between 148.0 and 149.4 mm. The expected strain percentage for the samples is between 0.0% and 1.2% strain. The reformer tubes are currently operating at very low levels of diametral strain. Long remaining lives are expected, with a maximum of 30% life consumed based on metallurgical assessment.
Stress rupture testing
A stress rupture test was performed on three tubes at 500 and 1,000 hours with stress selected to be 30 MPa i.e. double the mid-wall stress of 15 Mpa for the actual operating pressure and temperature, and 938ºC, the average tube skin temperature observed in the frequent TST survey.
It was concluded that:
- The life consumed is approximately 50% (compare to the time to rupture at 938°C). The remaining life for the “cold” section is 100,000 hours compared to 50,000 hours for the “hot” section at 938°C.
- Using the historical average data for either the bottom peephole level or middle peephole as a comparison (928°C or 938°C respectively), the remaining life is more than 100,000 hours.
- The reformer tubes should be capable of delivering another 10 years of service provided the operating temperatures do not exceed the historical averages.
Creep rupture calculation
Historical trends over operation time were reviewed for skin temperatures. The maximum average temperature was found to be 928°C for the lower peephole level. The maximum average found was 938°C which is a 10°C increase from the bottom. An additional 10°C buffer was added to the averaged historical temperature data to provide insight into the remaining life. All results are for remaining life expectancy across the reformer assuming 100,000 hours from commissioning.
Sensitivity analysis of remaining creep life
Sensitivity analyses were done to display the maximum percentage life consumed at a range of temperatures. Readings are based on the reformer middle peephole data as the temperature readings were higher than bottom peephole measurements. An additional 10% was added to the temperatures in the analysis to overcome the emissivity error while taking the pyrometer measurements.
Finally, the following conclusions were made:
• A low level of creep strain below 1% was measured in the reformer tubes by
ECT and laboratory diametral measurements. In total, 230 tubes were tested using ECT in 2021. Only five reformer tubes showed small indications.
• The through wall metallography confirmed no creep damage.
• A maximum of 30% of life consumed (as confirmed by the condition of the microstructure).
• The stress rupture tests showed a significant reduction in the creep life of the radiant section compared to the cold section.
• A maximum of 50% of life was consumed (as confirmed by stress rupture testing).
• The measured skin temperatures are significantly below the design temperature.
• With +10°C uplift, long remaining life was predicted using historical operating data.
• An average of ~3 start-up/shutdown cycles per year have not shown any creep damage on the ID of the reformer tubes.
• No significant reduction in life is expected due to current stop-start cycles.
• The remaining life of the reformer tubes under the current operating conditions is expected to be more than ten years.
- After evaluating the metallurgical properties, creep presence, estimated fractional life, the operating parameters fixed at 865°C as maximum and pressure of 18.2 bar.
- Accordingly, a full-fledged IOW program was established to monitor tube temperature on a daily basis. Since then, any excursion in operating temperature has been tackled and normalised immediately.
Process solutions for reformer tube integrity improvement
Capex and opex projects between 2018 ~ 2024 helped optimise SMR operations. The commissioning of an upstream LPG plant in 2021 stabilised natural gas quality and resolved air/fuel imbalance issues.
These changes reduced NOx emissions, improved heat distribution, and increased plant load from 103% to 106%. Energy intensity also dropped by approximately 1.15%, saving over $1 million annually. Major process solutions were adopted after the 2018 turnaround, mini turnaround in 2021 and the 2023 turnaround (see Table 2).
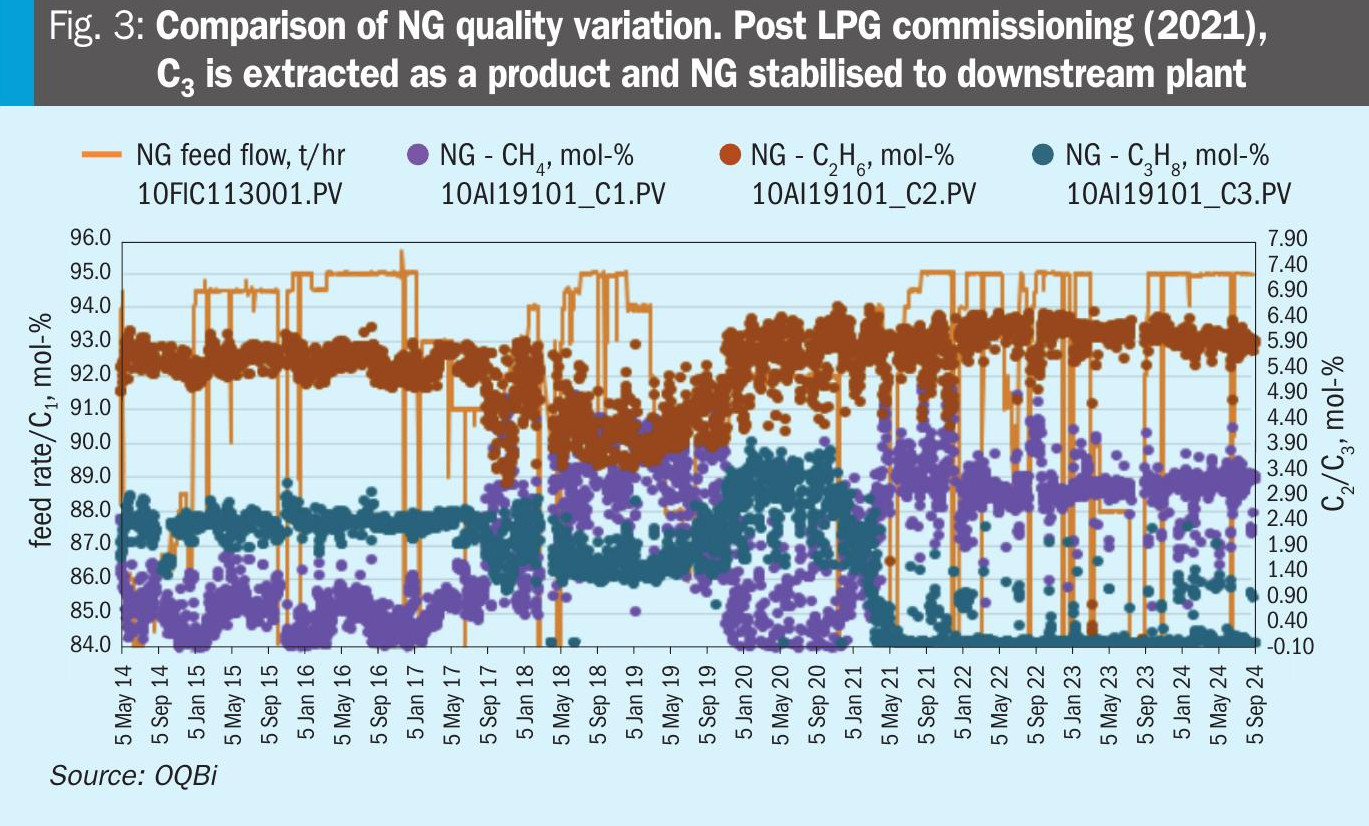
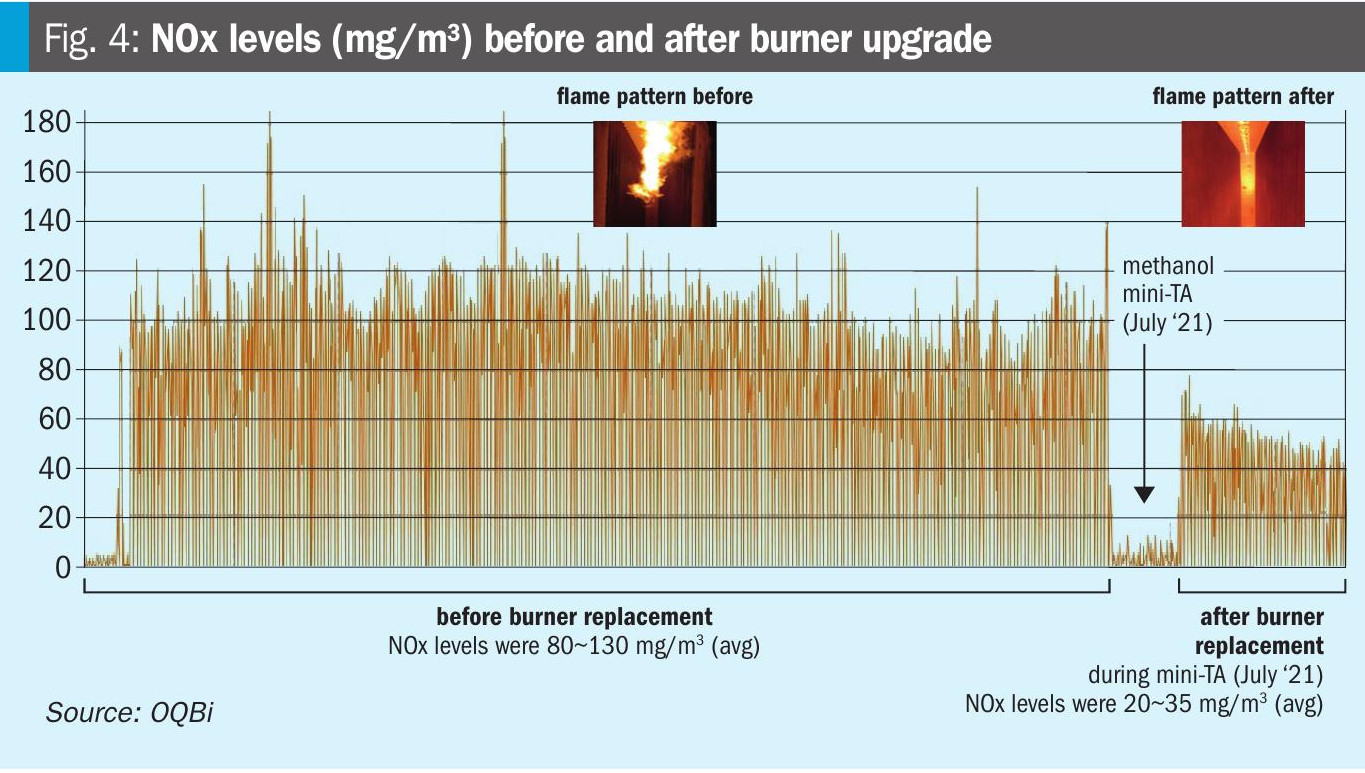
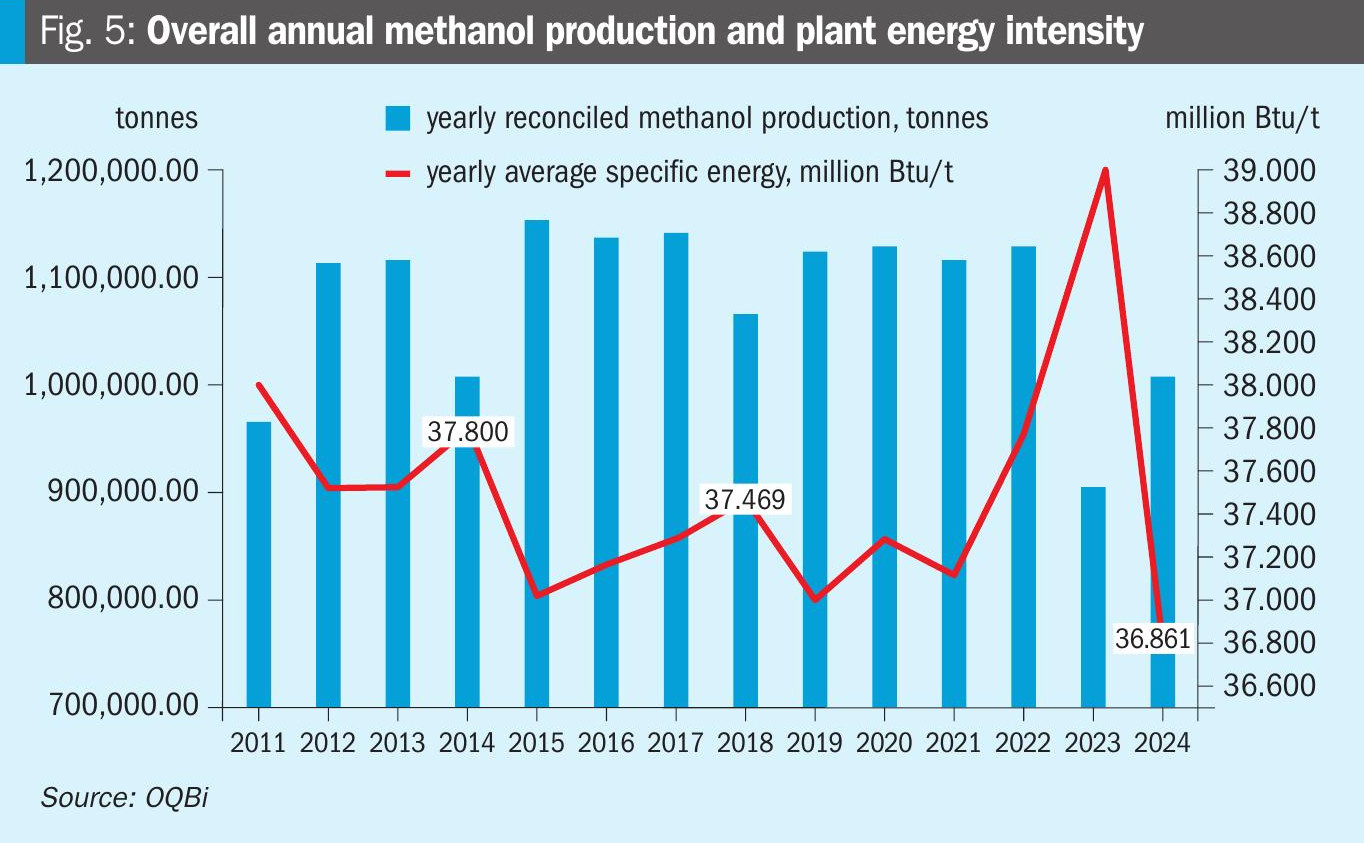
Challenges and action plan
Action plans have been put in place to address several remaining challenges.
Small eddy current indications in multiple tubes:
• outlet and cross header temperatures lowered to extend tube life until the 2028 turnaround.
• pre-planned tube inspections conducted at every available opportunity.
Significant temperature deviation might directly affect tube integrity:
• Integrity operating windows (IOW) program has been developed to monitor catalyst tubes, outlet pigtails, and cross headers;
• IOW limits narrowed further to prevent sudden excursions;
• advanced thermal imaging cameras deployed for tube skin temperature (TST) surveys;
• gold-cup tube surveys scheduled twice a year.
Burner tips choking due to throttled firing causing unstable flames:
• online inspection and maintenance procedures developed and implemented;
• daily and weekly visual surveillance initiated for flame pattern and hotspot monitoring.
TST survey may contain human and machine error:
• advanced thermal imaging cameras adopted for consistent TST measurement;
• biannual gold cup surveys added as verification;
• alternative peep-door inspection introduced.
Increased CO2 emissions post fuel configuration change (ammonia commissioning):
• scope and action plan finalised to achieve
• 25% reduction in decarbonisation targets
• 10% reduction in overall energy intensity.
Conclusion
Through advanced inspection techniques, improved process design, and automated monitoring, OQBi has significantly enhanced the operational reliability and lifespan of its SMR tubes, deferring costly tube replacement, improving plant efficiency and setting a strong benchmark for reformer management.